In this article I will present some information I discovered, which I believe is new information, that appear to extend and improve upon the existing scientific explanations about what caused the Younger Dryas, with some very profound implications.
While the numbers do seem to check out (see the middle part of this article for the exact methods and calculations I did), please be aware that I’ve done most of this work with ballpark figures and regular software available to non-academics. I’m just an embedded electronics systems designer, and I have no degree in neither space physics nor geology. I’ve probably done mistakes at various places, but I’ve done this honestly and to the best of my ability. If you find a mistake I’d appreciate the feedback, preferably in the form of a scientific paper reference, and it is likely that bits and pieces of this article get edited accordingly.
This article starts with a general popular-science introduction to the topic, and then switches to explaining my hypothesis, and finally ends up with some very specific numbers, trajectories, coordinates, dates and sequence of events. I provide a bunch of circumstantial evidence to back up my claims. The introduction is meant for normal people, so if you’re a researcher familiar with the topic, then just skip ahead to the hypothesis section.
My hope with this article is to attract the interest of scientists in the fields of geology, cosmology, climatology and archaeology, and give them the best information I can provide, so that they have the best starting point possible to formally verify or reject what I informally claim here.
What is the Younger Dryas?
It’s a period in time, approximately 12 thousand years ago, where it suddenly got cold and dry. It happened just after the ice age had slowly stopped and the world was slowly warming again. That’s when all of a sudden the temperatures dropped to ice age levels again. The period is about 1300 years long, and then the temperatures just as suddenly rose back up to where they would’ve been if the warming had been allowed to continue as it was going.

Weird.
During this period a bunch of animals went extinct, seemingly instantly during the start of the period. Mostly mammals and birds. Other animal populations were severely reduced or suffered massive range restrictions. Humans were left pretty okay, there’s just a small dip in population and signs that they radically reorganized themselves.
What is the scientific debate about?
People are arguing about what caused it. About ten years ago most researchers were reasonably convinced something had happened to the ocean currents, probably as a result of all the glaciers from the ice age melting, which in turn caused the Younger Dryas.
Then somebody wrote a paper about how a comet or an asteroid seem to have hit The Earth, at the same instant as the temperature started to dip, implying that it caused the dip, and they showed some evidence that put weight behind that argument. And in the ten years since then, tons of new research have been done on the topic. For every paper giving evidence for the non-impact theories about three or four papers have been published giving evidence for the impact theory. Like for example this one that details the only known example of a human settlement destroyed by a comet impact. Thanks to the recent results we can (as of 2020) confidently conclude that SOMETHING did indeed hit the earth 12 thousand years ago, and that it was probably pretty huge.
But we have no idea where it hit; we’ve found no crater that matches the age and scale of the event. There’s a big suspicious one at Saginaw Bay that we haven’t quite figured out yet, and another suspect in Greenland, but the Comet Research Group bet that it wasn’t only just one strike; they expect there must’ve been lots of fragments hitting the planet all over the place.
If you’re not familiar with the timeline of human history, let’s just say that’s the time when humans were just about to switch from hunting mammoths to growing crops. So we had stone tools, bone tools, wood tools, language, tribes, fire, clothes, jewelry, music, traditions, mythology, burial, hairstyles and everything like that. The north was still covered by glaciers from the ice age, but they were melting and retreating further north because the summers had started getting pretty warm. Meanwhile, the fields and forests were starting to get larger and larger populations of large animals.
That’s when it happened. Something hit the planet. The question is what and if it is the cause of the rapid climate change that happened immediately afterwards.
What kind of evidence have they found so far?
Digging in the dirt, at a certain depth we find a thin black layer. The black mat. It’s mainly found in North America, but can also be found in Europe, South America and Africa to a lesser extent. It indicates that the forests were burning. Lots and lots of forests were burning. Difficult to tell how many, but approximately a whole continent was on fire. So imagine something like 50% of North America, and 25% of Europe, South America and Africa respectively, and then you got the scale of the thing. All of them going up in flames at approximately the same time. We currently can’t tell if it took thirty years to happen or if it all happened in a single day.
In tons of various papers we have dated that layer to be placed at around 12780 B.P. plus/minus about 80 years (B.P. is measured relative to January 1st 1950). Just open any one of the research papers, they each reference their own date source, and this is the average of them as far as I can tell.
The researchers have also found that most of the planet was covered in a fine dust of platinum and palladium at the very same time. Very rare stuff on Earth, quite common on asteroids and comets. Even the parts of the planet that weren’t on fire got the platinum dust. We also find lots of microspherules and lots of nanodiamonds, that apparently form during such impacts.
We’ve also been able to tell that it was only the northern hemisphere that got colder. The southern hemisphere stayed the same, or even warmed a little. The “chilling effect” in the north appears to diminish slowly over a millennia until it suddenly stops. Furthermore, we’ve been able to tell that the summers in the north were just as hot as they normally were, which seems to tell us that all of the cold must’ve for some reason have been concentrated in the winters.
That’s pretty weird, because if something is big enough to mess up the whole northern hemisphere then that usually means it also messes up the southern hemisphere. It’s odd with such a selective catastrophe.
My new hypothesis
The cosmic impact guys have got it right, but they only have half of the story so far. They’ve seemingly neglected two key pieces of evidence:
- The effect came suddenly, lasted for 1300 years while slowly diminishing, then suddenly stopped just as quickly as it started.
- The northern hemisphere was heavily chilled during one season, while leaving another season seemingly untouched, and also leaving the entire southern hemisphere untouched.
As far as I know, there are almost zero natural mechanisms that does both those things in such a reliable fashion.
Except one thing. Planetary rings. Like on Saturn. But elliptical, not circular. If you tilt the rings just so, and make them elliptical like just so, then they shade only a specific hemisphere of the planet during only a specific season. And on the other side of the planet it forms a giant mirror in the sky that gives the other hemisphere a little bit extra sunlight during another season. They also appear very rapidly and disappear very rapidly.
Yes, I’m saying that The Earth had rings 12 thousand years ago. Stay with me, because there’s a whole bunch of circumstantial evidence coming up. Let’s just go over the general overview for a few more minutes. The rings would have been angled pretty much exactly like in this picture:
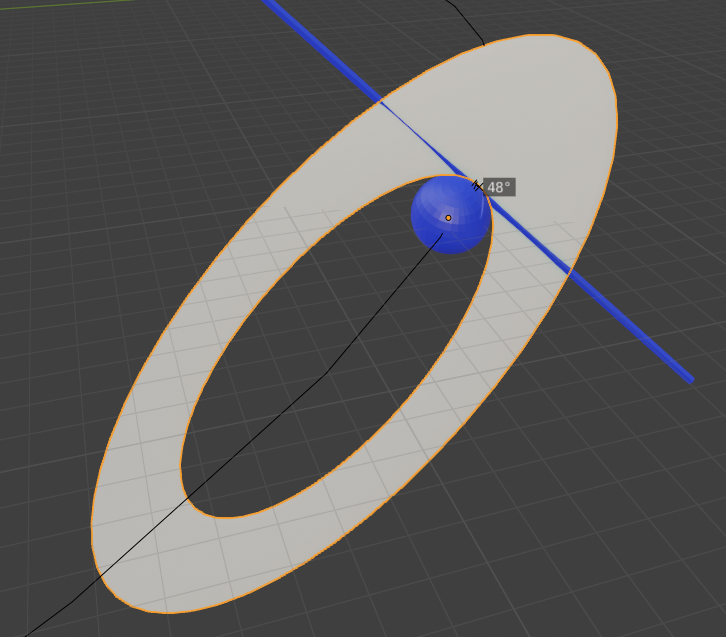
Their lowest point, the perigee, would have been just 100 km above the surface of Earth. Because that’s where the air starts. Their highest point, the apogee, would have been about 1/3 of the way to the moon. Because beyond that the moon has enough power to mess things up.
I have not yet found any scientific source for the claim that they both appear and disappear very rapidly, but I’m so convinced of this fact that I’m letting it stay even without a source, confident in my own explanation given later in this post.
And why did we have rings? Well it’s because the end of the world almost happened. We’ll get back to that in the evidence section.
First let’s talk about how do you get rings! You need three things: You need water ice (A) and you need to make it move at orbital velocity (B) and then you need to circularize its very elliptical orbit (C).
C is done by accidentally having the moon at the right spot at the right time. A and B can be accomplished by either:
- Impacting the Earth with an ice comet at an extremely shallow angle.
- OR
- Almost impacting the Earth with a fragmented ice comet at an extremely shallow angle, just barely missing the planet.
- OR
- Impacting the Earth with a rock asteroid at an extremely shallow angle, and have it hit a very thick glacier sheet.
Or you can do a mix of them: having a fractured ice comet where some fragments of it impact a thick glacier sheet while some other fragments misses the planet. Either works!
But all of that was just my hypothesis. Was any of it plausible? Could it have happened?
How I validated its plausibility
To start with I needed a guess; a guess that I could use as a starting point and improve upon. So first I drew (in the free 3D modelling software Blender) how the rings must’ve looked to produce the desired sunlight-shading and sunlight-mirroring effects from different angles. I quickly found that they would have to be pretty elliptical, and they would have to be tilted a specific way. This sketch enabled me to estimate maximum and minimum values on the latitude of the impact location (45 degrees north, plus/minus 15 degrees). It also enabled me to estimate minimum and maximum values of the course of the incoming object (either course 230 plus/minus 20 degrees, or heading the exact opposite way). I estimated these values by squinting really hard at the screen.
Since the summers were as hot as normal I also knew that the shade had to have been placed on the northern hemisphere somewhere between august and may. But it was very probable that the shade was placed somewhere between November and March. This gave me minimum and maximum values for the celestial direction that the object could have come from.
These values helped me narrow down the search area of the heavens that this object may have come from. Here’s exactly how I did that: I placed a green rectangular bar, angled with the expected course, at the central latitude (45 deg north) and two other similar red bars at the extremes (30 and 60).
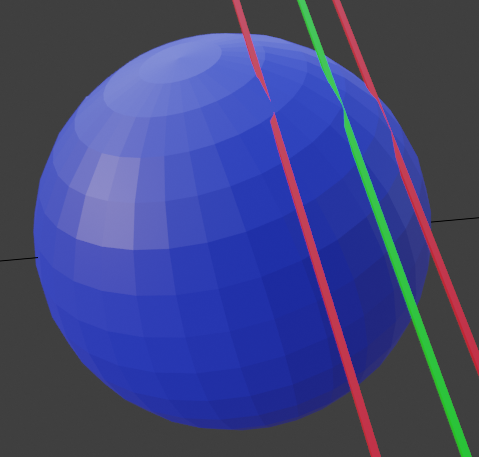
Then I rotated the planet around its axis. Imagining the bars being laser pointers being swept across the sky painted two wide bands; one on the northern hemisphere and one on the southern hemisphere. These were the permitted areas, with the center strip of the bands being the most likely.
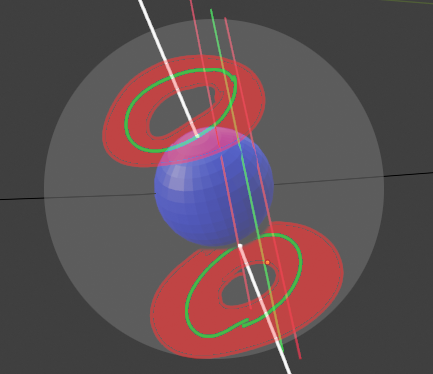
But thanks to knowing that the summers stayed as hot as normal, and it seemed that only the winters got really cold, I could exclude some sections of those ribbons. That’s because a strike at the wrong time of day would make the resulting rings angled the wrong way, and would chill the wrong season. So thanks to that I could discard sections of the bands, and place heavy probability at a specific section.
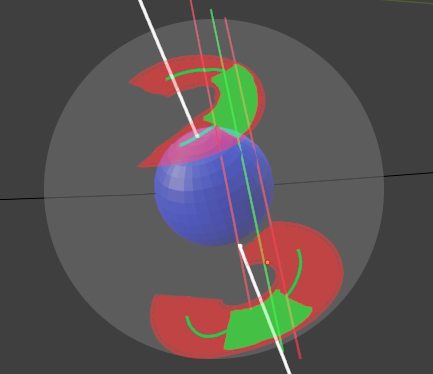
By the way, if you don’t understand how it can be so obvious to me how the rings would be angled from each attack vector, then you need to play some Kerbal Space Program. It’s kindof tricky to explain orbital mechanics in words in a single paragraph, so the quickest way for me to tell you how I did that part is just to tell you to go play the game. All of this stuff will be obvious to you after a few evenings of playing it.
But stated briefly: The point of where the impact happened necessarily also is the lowest point of the resulting elliptical orbit, and the rings necessarily then curve around the gravitational center of the planet. That’s all. There’s no other possible way they can move. And that gives you the angle of the rings.
After I had the most suspicious areas of the sky marked out, I then adjusted the orientation of the starmap to account for how it had changed since these prehistoric times. Specifically: I used the free software Stellarium, and re-winded the clock 12 thousand years (to 10910 B.C, which is the guesstimated starting year of the Younger Dryas). After doing a sanity-check on the rate of precession of the Earth axis, which the program passed with correct numbers, I gained confidence that the makers of that program knew their stuff. Therefore I trusted their rendition of the prehistoric night sky.
Next, I used another key insight. If an object of this kind passed this way and did these things, then it would definitely have left a trail of debris that would be visible today as an annual meteor shower.
So I plotted the location of all the major annual meteor showers on the sky and checked if any of them was inside the search area. And it turns out that most of the showers are outside of the area entirely, while a single one of them is suspiciously close to the center of the northern search area:
The Perseids.
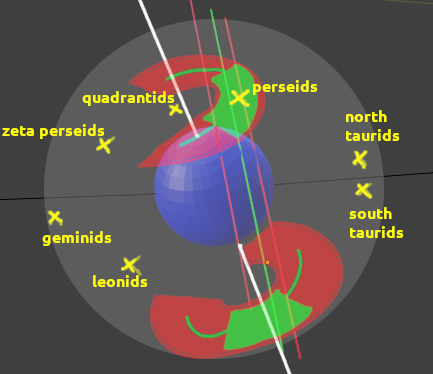
It is so suspiciously close that I feel safe in assuming that The Perseids radiant indeed was the origin of the object, which then locks down multiple unknown variables since the location of the radiant is known to a very high degree of accuracy. Coupling that with the prehistoric sky rendition within Stellarium and the angle measurement tools within Blender, and a high-resolution starmap texture downloaded from NASA (imported into Blender as a skybox texture), that enabled me to calculate all sorts of things with very high accuracy. Which I did. Without squinting.
This gave me the month and day of the event, an exact impact vector relative to the ground and an approximate latitude of the impact. The month was February. The day was 15. The latitude was at 45 degrees north +/- 15 degrees, and the course for that latitude was 228 degrees (measured clockwise from true north) +/- 1 degree. The course varied with latitude according to some mathematical formula I didn’t bother producing with mathematics; instead I would estimate the result of the formula by simply measuring angles directly in the 3D model.
Remember how I said that we must circularize the orbit of the debris, by accidentally having the moon at the right spot? Well now, knowing the time and location of the impact, I used Stellarium to tell me where the moon was at that point in time. Once I found it, I needed to know what effect its gravity would have. So I used the software Kerbal Space Program to check what kind of effect that arrangement would have on debris moving in the way I had calculated. I put a spacecraft into an elliptical orbit that looked like the orbit the initial debris would’ve had. Then I waited until I was at apogee (furthest from Earth and closest to the Moon) and fired thrusters to accelerate into the direction the moon was at during that time. That would simulate the gravitational effect of a moon at that location. The results I got was that the orbit was circularized, and tilted to become slightly more polar. This meant that after a single orbit around the Earth, despite the first lap being down in the atmosphere, the second lap would be higher in the atmosphere, and almost missing it entirely, and thus doing much less aerobraking. The aerobraking meant that on the second lap it wouldn’t get quite as close to the moon as on the first lap, making the effect diminish slightly. Probably by the third lap or so, the orbit would be clear above the atmosphere and do no more aerobraking. Since the orbit of the rings was only about 1-3 days or so, that meant the moon was still in almost the same location on the second and third lap. The effect would’ve diminished to almost nothing by the 5th lap. The rings would have started out extremely elliptical, but after a few laps the apogee would have dropped significantly and made them more circular.
So in other words, I used a space physics simulator and found that the moon was indeed in the correct spot to circularize the orbit of the debris!
That gave me more confidence that I was on the right track.
Going onwards from there, I figured there might still be some large objects remaining within the Perseids meteor swarm, which could be the remnant of the impactor, so I checked if that swarm contains any known large object. And it turns out that indeed there is one. A very large comet. Upon checking where it is now and its orbital period, the numbers matched up frighteningly well.
To do this calculation correctly, we need a good signal for exactly when the platinum was deposited all over the planet. I take this figure from the paper where the platinum was first published, because it has a very sharp peak, and by examining where the peak is in the graph I see 12888. They do not write “B.P” but I guess that’s what they mean. If we use that as the start of the Younger Dryas and calculate the interval from there until the next time the comet will pass close to us (Aug 5, year 2126) we get a length of 13064 years. If we divide that by the comet orbital period (130,48 years) and get something close to a whole number, then that means the comet was close to crossing the orbit of The Earth at that time. And what number do we get?
100,12.
That means that the next time it passes in close proximity of The Earth, it will have been very close to 100 orbital periods since the platinum peak. That extra 0,12 translates to 15,9 years away from my initial guess of 12888 B.P, placing the impact at 12872 B.P. (10922 B.C).
Assuming that the encounter happened at 45 degrees north (i.e. the center of my guess), I edited the 3D model to account for these dates, and the angle that the comet would have arrived at, and checked what kind of ring would be produced. Everything north of 50.0 degrees latitude, approximately the southern edge of Denmark, would have been in permanent shade for half a year, every year, for the whole lifetime of the rings. The places more south than 50 degrees would also see some shadow, but only for shorter periods of the year, and usually just fractions of each day. Meanwhile, the places south of the equator would see almost no shade at all; they would just be shaded for an hour every day during three weeks in the spring and three weeks in the fall.
Here’s a table over roughly how shaded the planet would be during the different seasons. The numbers are given as percentage of each day that is in shade. I estimated this by rotating the 3D model and looking at it and visually estimating how much was shaded from each angle (i.e. not very exact at all, but at least they were estimated from a proper 3D model rather than just my imagination).
Southern hemisphere | Lat 0-50 north | Far north (lat 50+) | |
January | 0% | 25% | 100% |
February | 10% | 10% | 50% |
March | 5% | 5% | 5% |
April | 0% | 5% | 0% |
May | 0% | 0% | 0% |
June | 0% | 0% | 0% |
July | 5% | 5% | 5% |
August | 15% | 25% | 50% |
September | 5% | 25% | 100% |
October | 0% | 10% | 100% |
November | 0% | 5% | 100% |
December | 0% | 5% | 100% |
I then checked that result against the impact crater that researchers have found the most suspicious (Saginaw Crater, coupled with the Carolina Bays and the Nebraska Bays). First of all, the latitude of the crater is at 44 degrees north. So it’s only off by a single degree from the center of the most probable latitude in my estimate. Secondly, the course was off by only 6,5 degrees (the crater axis has the course 221,5 and the Perseids has 228). And looking at the 3D model, it is clear to me that the difference is in the exact same direction that gravitational bending and atmospheric drag would have pushed the trajectory, but I don’t know how to calculate how much. If I had to guess, I’d say the trajectory would be bent by about 5 degrees, thus leaving approximately only 1-2 degrees difference to the Saginaw Crater axis.
I can’t tell for sure if the crater is indeed related to the rings, or if it is a completely separate event, but I’m pretty excited about how well everything matches up!
In conclusion, the very close match between the location of the Perseids, the location of Saginaw Crater, the angle of Saginaw Crater, the location of the moon, and the orbital period of the comet, and the date of the platinum deposit on the Earth, and the correspondence between my resulting rings and the climate effects we’ve seen in the historical record, have made me quite confident that my results are worthy of being written down as a public post on the Internet.
Volume and mass calculations
I tried finding proper scientific articles about how much water is in a typical mist, but the one I found was overly math heavy. So I found another article instead which made an easier statement: “1/2 gram of water per cubic meter of cloud”. And they were talking about opaque cumulus clouds.
Another way to state that number would be: 1/2 gram of water per square meter of area we want to make opaque. That way it doesn’t matter how deep the cloud it. Thus we can disregard the thickness and just calculate areas. But we only want to make it semi-transparent so let’s make that 1/4 grams of water per square meter of area.
The rings in my 3D model above have been modeled as follows (units are in diameters of the Earth):
- Outer perimeter major axis is 10.3
- Outer perimeter minor axis is 3.9
- Inner perimeter major axis is 6.15
- Inner perimeter minor axis is 2.3
The resulting area of the ring surface is: 1.75 x 10¹⁰ square kilometers.
1/4 grams per square meter gives us: 4.36 x 10⁹ tons of water
Which can be contained in a cube of of ice that is 1633 meters to a side. Considering my ballpark estimate for the diameter of the main impactor is 5 km, that would make the rings about 3% of the volume and weight of that impactor.
In other words, the volume and mass of the rings is completely consistent with the rest of the hypothesis, and leaves plenty of room for plenty of ice bergs to be mixed into that mist, continually replenishing it. It also leaves plenty of room to make the rings denser (more opaque), in case the “shadiness” of my proposed density is not strong enough.
But at least we have clearly established a bare minimum size of the comet fragment: 1600 meters cubed. In order to also fit in the replenishing ice bergs, I’m expecting a total mass of at least twice that.
Specifics of the close encounter
It appears that one or many broken-off chunks of the Comet Swift-Tuttle encountered the Earth at 60 km/s, coming in at an angle of approximately zero degrees (depending on how you measure it) at approximately 45 degrees north latitude, with a course of 221,5, somewhere between Eastern Europe and Western North America. It is not certain that the pieces touched the ground; they may have simply coasted through the atmosphere and then departed. The whole encounter, from entering the atmosphere until departing again, took about 50 seconds.
It happened in the year 12872 B.P., during early spring, sometime during the night of either February 14, 15 or 16 of that year (calendar adjusted to match the equinoxes and make sense to normal humans). Most likely it was February 15th.
Somehow, ice ended up in orbit at the same time going in the same direction as the comet, but slower. I can’t quite tell exactly how that happened. I talk about the various possible scenarios in the section “Methods to get orbital ice”.
Since we see evidence of so many airbursts happening simultaneously all over the planet it is fair to expect that the broken-off chunks were not alone. There is a fair chance that the Comet Swift-Tuttle itself was not very far above. I believe it was either touching the atmosphere itself with its belly, or at least it was passing by the Earth somewhere immediately outside of the atmosphere. I believe this because all of the impacts seem so have happened at the same time at the same location, rather than being spread out in a long line across the planet surface. If the comet had broken off the chunk during the last passage past the Sun (130 years prior), then the parts would’ve been much further apart, and they would form a long line across the planet. So it seems to me that due to how they all appeared at the same time at the same location, that the fracture likely happened just an hour or so before arriving at Earth, and that it therefore happened due to Earths gravity.
The vast majority of those pieces of ice would have been between ice cube and ice house size, of which most would produce “airbursts” (see the section on airbursts in the end for more detail on this). They would look a bit like nuclear explosions up in the sky. Those explosions would have been able to heat things up from afar, simply through the light they emit. If there were many enough happening at the same time it would seem as if the entire sky over the entire continent glowed like a nuclear bomb, thus setting the ground on fire without even touching it. Most of them would have exploded (or simply burnt up into vapor) on such high altitude that the shockwave (and sound) of the explosion probably wouldn’t even make it all the way to the ground. So for the vast majority of the incoming ice pieces the intense radiated heat and light would be the only effect on the ground. Just a few pieces would have physically made it to the ground, in particular the largest ones. Perhaps only the single largest one; Alpha.
The Comet Swift-Tuttle still exists today, and it is called “the single most dangerous object known to humanity”. Because it is big, it often crosses the path of Earth, and it is in retrograde orbit. That last detail is important; it means it is going the opposite way around the sun compared to The Earth. That means it is going terribly fast relative to Earth. That means if it hits us it will be a very bad day. In fact, it carries 26 times more energy than the little puny rock that killed the dinosaurs.
So yes, I’m saying that my model indicate that 12k years ago all of humankind came so close to dying that it’s not even funny.
If Saginaw Bay is related…
…then we have a bunch of extra information we can use. This section details the extra information we can extract if that happens to be the case. Please note that this relation is not required for the hypothesis. It is just an optional bonus.
Aside from then knowing the exact location of the event, we also know that the impact happened at 5 a.m. at night, in the timezone GMT-4, January 15 in the year 12872 B.P..
The main impactor, let’s call it Alpha, then would have come in over Newfoundland and Labrador, crossed over Quebec, touched ground in Saginaw Bay. That location, at the time, was covered by a 1-2 km thick layer of glacier ice. I have found it difficult to find scientific papers that detail what happen for extremely large pieces of ice that impact The Earth, but two scenarios are conceivable:
- There could be a minimum size, above which an ice impactor reaches the ground without airbursting. So really large things might reach the ground.
- There could be a solid core within the comet fragment, either stone or metal, which survives the airburst of the ice around it. So while all the water is shed in the atmosphere, the hard core reaches the ground.
If a part of it reached the ground, due to any of the above reasons, then this glacier ice it landed on would have been sprayed all over the place. Any high-energy leftovers from the encounter would have would have continued its flight over Michigan, Illinois, Missouri, Oklahoma, Texas, and then finally left the atmosphere entirely over Mexico.
If Saginaw Bay is related to the incident, then that makes Alpha a significant part of the original comet, and it places Swift-Tuttle in a terrifying position, just barely grazing the atmosphere. If so, then the whole sky over North America should have been filled from edge to edge by incoming cosmic ice bergs and ice cubes. And there should have been a fair number coming down over Europe and north Africa as well. Here’s a view from the comets perspective as it would have approached us:
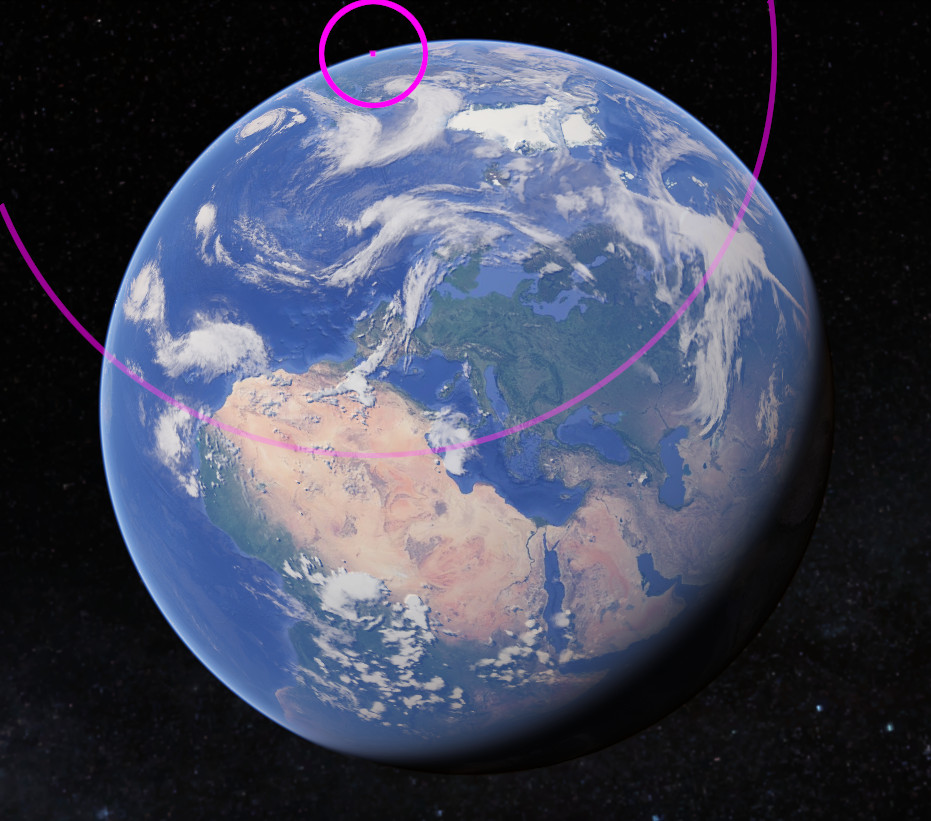
As you can see, that was pretty dang close.
The dot indicates the point at which Alpha would have strafed Earth (i.e. Saginaw Bay). Since Swift-Tuttle didn’t impact the planet, we can assume that it passed Earth somewhere in that black-sky region of the purple circle in the above picture. If we’re talking about the inner or outer purple circle, I leave up to you. I don’t know, but I’m betting on the inner one. All parts of the Earth that are visible in this picture would possibly have been struck by fragments, while the ones not visible had no risk of being stuck. So if we find any more craters of the event, they must be placed somewhere in the above picture.
In the above picture, the Earth is currently moving through space towards us, and towards the bottom left corner of the image. So you see how the comet would have almost hit us from straight ahead, kind of diving down on us from above.
The debris field from this impact would most likely be spread out like the red area in this image I just sketched up, based on what’s viewable within the circle in the above picture, plus the splashdown cascade pattern (with butterfly wings!) that the touchdown of Alpha would have caused. This is the area in which we should be able to find small pieces of Swift-Tuttle.
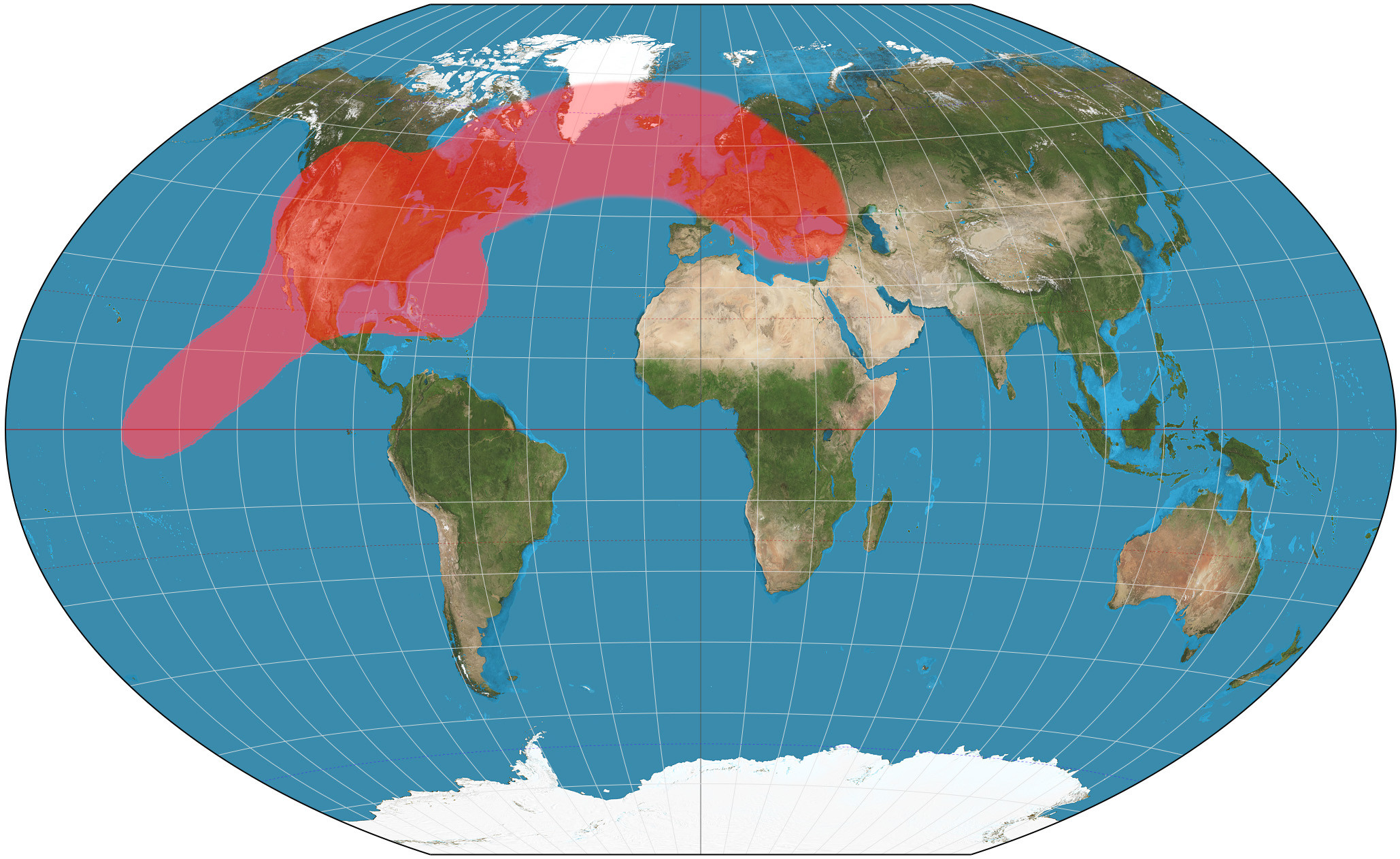
We can use this hypothetical debris field, and compare it to the debris field we’ve actually found from the Younger Dryas, and if they match then that’s yet another piece of circumstantial evidence pointing towards this location.
The Rings of Earth
After we got supersonic ice into space, we need to have the moon in the correct spot by pure accident, which is like a 10% chance (and happened to be the case at the date I propose). If the moon happens to be at that spot, then it will perform a gravitational slingshot on the ice chunks, speeding them up a little when they’ve reached the outermost part of the ellipse. That circularizes the orbit. If it hadn’t done that, then the ice chunks would’ve simply plummeted back down into the Earth after a single orbit.
But how do ice chunks become a misty disc?
In space we have vacuum, so ice chunks sublimates (vaporizes) into microscopic water droplets (actually atoms) that eject themselves from the ice chunk. And in space we also have intense solar irradiance, which speeds up that process. So what starts as just a swarm of giant icebergs have within just a single year or a single decade vaporized into a even mist of tiny snowflakes interspersed with icebergs that are slightly smaller than the ones we started out with. The icebergs will stop diminishing in size (evaporating) once the orbit have been saturated with the mist and a solidly visible ring has been formed. This is because they’ll reach steady-state, where the ice bergs receive as much water atoms from the mist as they donate to the mist.
The rings are not a perfectly flat disc. It is more like a doughnut that you’ve stretched out a lot and flattened.
In the end, by my estimates, the rings extend to about 1/3 of the way to the moon on the apogee side, while skimming just above the atmosphere on the perigee side.
As the Earth revolves around the sun, the rings stay angled the same way, as shown in the below image, which is taken from above the solar system looking down. The size of the objects are exaggerated and not to scale, only the angles are correct.
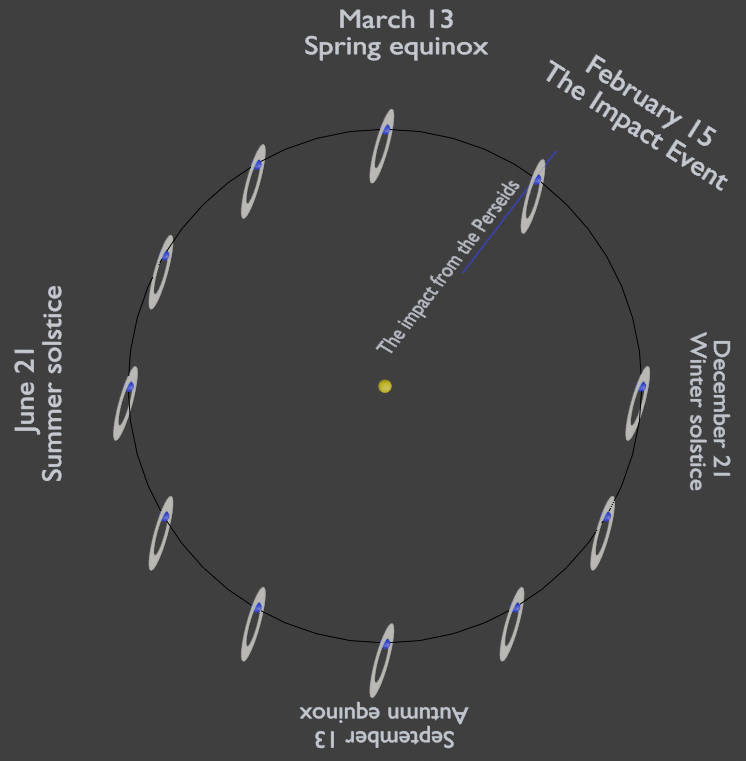
Here’s the same 3D model but from an angle:
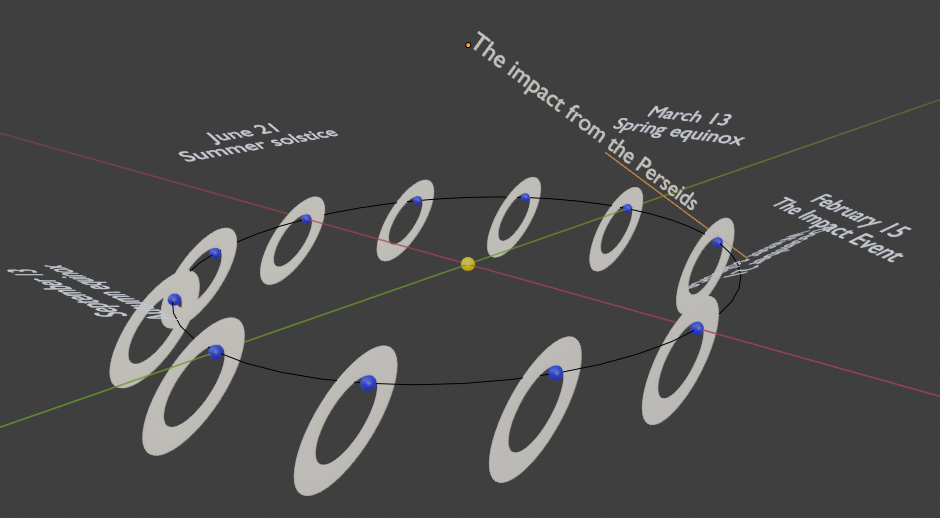
Here’s the planet in the middle of winter, as seen from above:
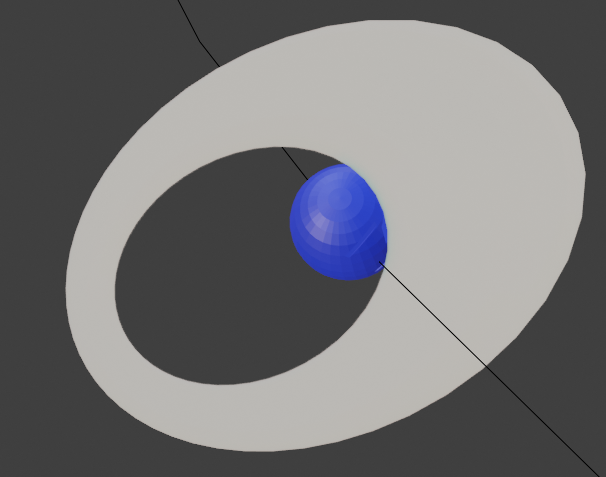
And here it is as seen from the sun at the same date:
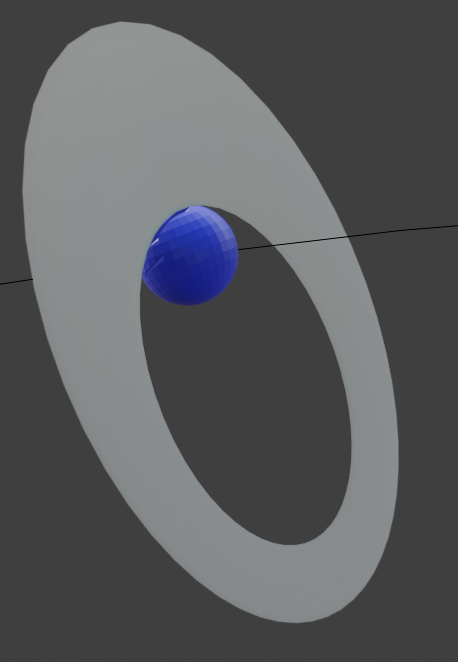
As you can see, there’s a significant part of the north hidden behind the disc. Compare that with how the southern hemisphere looks during the southern winter:
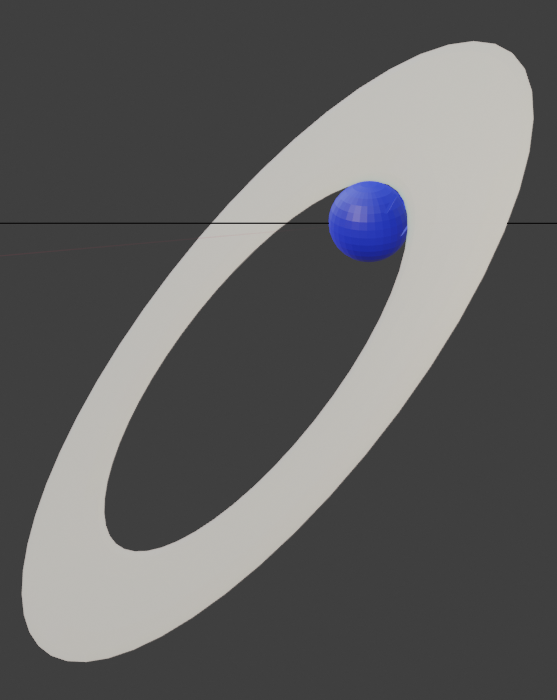
As you can see the south isn’t shaded at all! And while it is summer in the north, the disc is behind the globe, so no shade there either.
The rings will be milky white, and look like a milky white road across the sky. When the rings passes nearly in front of the sun, and nearly shades you, then you will see a rainbow halo around the sun (ice crystals do that, ask any polar scientist, they see sun halos all the time). If you’re in the shade of the ring then the sun will look dimmer. I’m not sure how much dimmer, but I don’t think it’ll block all of the sun. I expect you to be able to see through this, like a mist.
When seen from the ground the rings will look different depending on where you’re standing and what season it is and it even varies depending on what time of the day it is! Sometimes its a vast circle filling half of the sky. Sometimes it’s just a thin vertical line leading straight upwards from the horizon. It is very likely that some people started using the different appearances of the ring during different parts of the year as a kind of obvious calendar. In the far north, at some hour every day (and sometimes during night) it will look like a distant arching bridge, that leads from the ground at the horizon to the sky above. During some seasons in the north the “bridge” will go close enough to the sun for an hour or two, to make it appear to be made out of some rainbow material:
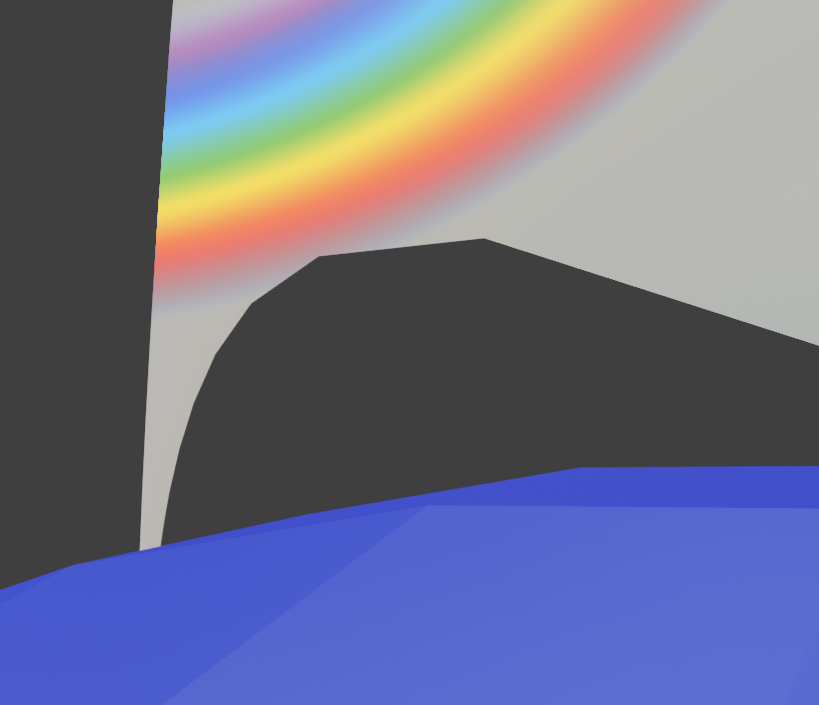
Since I cannot tell (until I get help from cosmologists and climatologists) how dense the mist was, I don’t know if during the majority of the lifetime of the rings they were as dense as a cumulus cloud. Opaque. In that case, there would be no rainbow effect until the very last years of the ring, where it would finally be so thin that the sun could penetrate through it. Because the rainbow effect is only produced when the mist is very faint and thin. So even if it didn’t look like this during most of the millennia, at least it would look like this during the final years before disappearing, at around 8500 B.C.
I’m assuming that over the next thousand years after the impact the moon perturbs the rings, and they’re gradually slowed by the close passes of the atmosphere, so eventually all the ice in orbit crashes into the Earth. But this time they’re only moving at regular orbital velocity, which is not very dangerous at all. The bulk of the dangerous energy was already dissipated in the original incident that produced the rings. So the remaining icebergs are not so dangerous. At least not on a global level. It’s just an iceberg falling from the sky. It might form a small crater, if it doesn’t break up on the way down, and it’ll add a tiny amount to the ocean water level, but I don’t think the amount will be noticeable.
I mean, we have seen melt-water pulses in the time after the incident, and I guess they could be ice bergs coming down from orbit, but I’d really bet against that. As far as I can tell the melt-water pulses we measured are way too big and the ice bergs in orbit would be way too small.
I have not yet found any source on the behavior of orbital mist produced by sublimating ice bergs, so the following stuff is just my guess about how I think such things should work:
The icebergs in orbit replenish the mist. We don’t need many of them to keep the mist fully saturated, but once the last iceberg crashes down, the mist is going to dissipate quickly afterwards. So we shall see a gradual linear decline of the ring density, with small shooting stars every night, and then finally a very sudden drop, once the last large ice berg crashes into the planet. After that there’s nothing left to replenish the mist, so it is quickly blown away by everything from moon orbits to solar wind. This should be reflected by the temperature data, that should show a linear increase in northern hemisphere temperatures, followed by a very sudden return to the normal high temperatures.
Human symbolism
Let me make a brief step away from cosmology and physics, and talk about humans for a moment.
If such planetary rings indeed existed, then they existed during the recent human history. Thus they would necessarily be recorded in human mythology and art. Somehow. Somewhere.
I tried to imagine how I would graphically depict the rings in artwork if I had only ever seen them from below. Like how ancient humans would have seen it. Here’s my best guesses for what such artwork would look like.
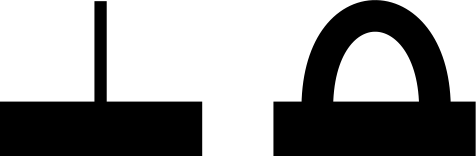
- Leftmost image: A symbolic depiction of what the rings would look like during the most special moment of the day: when the Earths rotation brings the view of the person to be directly below the rings, so they’re viewed edge-on. They would look like a perfectly straight line emerging from the horizon, heading up across the sky. This is the 1-minute-long moment during each day when everybody would look up at the rings, because it is a very short moment during which the rings look really peculiar.
- Rightmost image: A symbolic depiction of what an artist would draw, if he was not trying to draw the special moment, but rather trying to come up with a symbol for the rings that couldn’t be misinterpreted as anything else. He would try to make sure it couldn’t be misinterpreted for a setting sun, by intentionally making the arcs non-circular. A pedagogical artist. An artist of the people.
All in all, I would expect 90% of the artwork to use the rightmost image. The leftmost one would be used in the few cases when making an astronomical almanac and you wanted to indicate a precise time of day.
How airbursts work
Now let’s get back to physics!
A chunk of ice going 60 km/s through the atmosphere will glow. A lot. For reference, the 2013 Chelyabinsk meteor was going at a sedated 19 km/s. You’ll sense the heat on your face from the ground. It can keep going like that for a while, until it accidentally fractures.
Typically, incoming meteors explode in mid-air. But some stuff is obviously able to travel at orbital speeds and then enter the atmosphere without exploding; just look at the spaceship capsules that enter with their ablative heat shields underneath. So what’s the difference?
To get an intuition for this, imagine throwing a chunk of solid ice, and compare that to throwing a fistful of dust. Solid ice chunks can punch through the air, but dust cannot. It is simply too much air resistance for dust. Liquid water going at supersonic speeds immediately turns into water droplets, which is basically dust. Dust immediately aerobrakes against the air. Stuff aerobrakes harder the smaller it is. The surface area is very large compared to the mass and volume.
So if a meteoroid chunk of ice fractures into two chunks, that’s prooooobably fine. They’ll probably continue flying through the air like meteors. But if a chunk fractures into lots of small pieces at once, then they’re suddenly aerobreaking so fast that their backsides are crushed against their fronts, turning all the small pieces into ice gravel. The ice gravel then aerobrakes even harder, crushing them to fine ice dust, which then brakes even harder. This is a runaway process that basically stops the meteor in mid-air in less than a second, and all that energy gotta go somewhere. All the incoming kinetic energy is converted into heated air and steam in a fraction of a second. From the outside it looks like a nuclear explosion.
The trigger doesn’t have to happen due to an unpredictable random breakage either; since the atmospheric density increases further down, the aerobraking force will increase accordingly as it plummets deeper into the atmosphere. The first fracture typically happens when the aerobraking force exceeds the material strength of the object. Due to the runaway process, an immense number of fractures typically follow within a split second of the first one.
Conversely, the larger an object is, the less it aerobrakes in relation to its size. So there is a certain size above which the reaches the ground. So the airbursts I’m talking about in this hypothesis will have been ice cubes and ice buildings. But not ice mountains.
Methods to get orbital ice
To produce planetary rings, we need ice in orbit that moves much slower than the comet originally did. We can accomplish that by multiple methods, and I’m not entirely sure which of the following scenarios are most probable. Perhaps you can help me figure it out:
Scenario A: Alpha did not touch the ground. It merely aerobraked in the atmosphere and plopped out the other side, relatively unscathed. In this scenario, the rings were formed by the body of Alpha itself and involved zero glacier ice.
Scenario B: Arriving to the thick glacier sheet at a nearly horizontal angle Alpha (or its solid core) shoveled ice in front of it like a bulldozer, and also to the sides of it like a snow plow, until it dug down deep enough to to encounter bedrock. It traveled for a considerable distance horizontally into the glacier before coming to a stop. The wall of “bulldozed” ice in front of it was ejected at orbital velocity and departed the atmosphere. There will be a significant circular crater that formed due to this. In this scenario the rings were formed mainly by this glacier ice, and perhaps (but to to a lesser degree) by the debris from Alpha itself.
Scenario C: Like in scenario B, but after plowing through the ice sheet Alpha (or its solid core) did NOT come to a stop on the ground. It refused to stop at the bedrock and just ricocheted (bounced) on it like a bullet strafing a metal plate, and came back up, now with swarms of supersonic ice bergs hitchhiking along with it. In this scenario the rings were produced by both Alpha itself and the glacier ice that it stole from the planet, in approximately equal amounts. Please note the critical detail here: in this scenario Alpha did not come to a stop, which means there will not have been a massive explosion, and there will not be a massive circular crater. There will be a minor crater (minor for an event of this scale, but still kilometers big) and it will be very elliptical (not circular), perhaps even line-like, and quite shallow compared to the size of the event. The debris from the glacier will have gotten orbital velocity not by getting impacted by an explosion, but rather by getting impacted by the momentum of Alpha (and the thick wall of supersonic pressurized steam ahead of it).
As you can probably tell, scenarios B and C require Alpha to be HUGE (or else it would be vaporized against the atmosphere like a bug against a windscreen). After reading a few research papers about comet impacts I guess this would necessarily make Alpha something like 5-10 kilometers in diameter. Meanwhile scenario A would only require Alpha to be about 2km in diameter (see previous calculations on the mass of the rings). In either case it is fair to expect it to have been a sizeable chunk of the parent comet. Swift-Tuttle itself is 26 km across nowadays, and must’ve been quite a bit larger back in those days. So my current guess is that Alpha was a 5 km breakaway from an originally 35 km Swift-Tuttle.
Like I said, I can’t yet quite decide which scenario I consider most likely. If you know your comet impact theory then I’d love to hear your comments on my reasoning about scenarios A, B and C.
Carolina Bays
Did you know North and South Carolina are jam-packed full of oval rings? Go to Google Maps and have a look at satellite photos! Below is an example, I think I can see 10 ovals in just this little area around Dillon and Lake View.
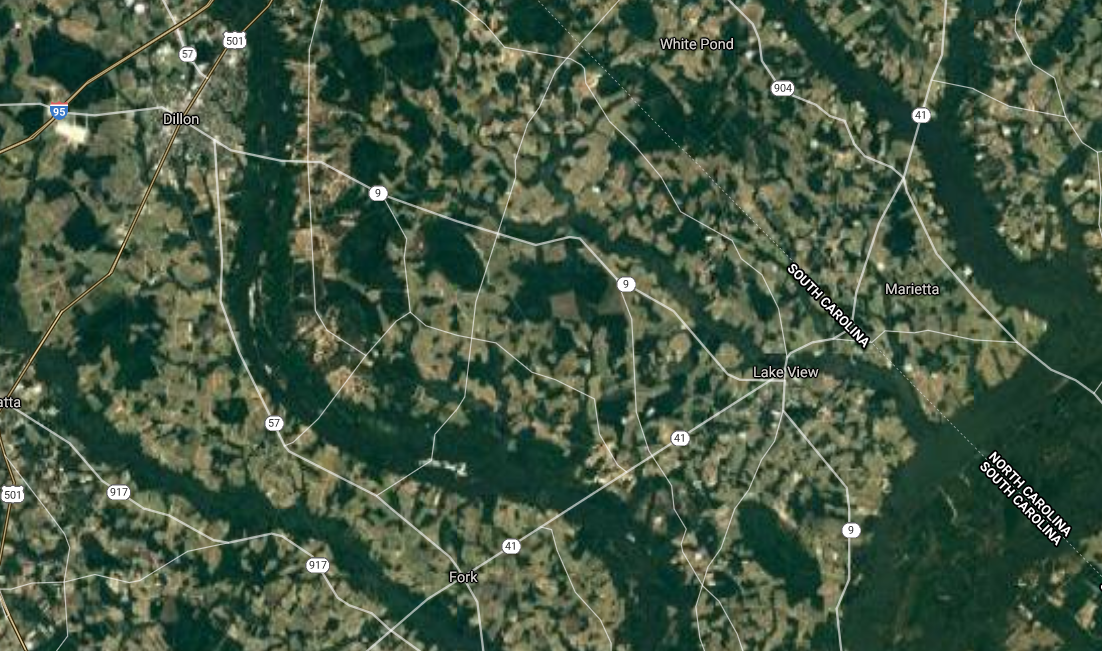
And here’s what those areas look like when scanned with lidar. As you can see, for every circle you can see with a regular camera, there’s 10 or 20 you can see with lidar.

The common explanation for the Carolina Bays is that they’re the remains of small pools that were shaped by wind over a long time to form perfect ovals. Compare then to the thermokarst lakes of Siberia, the next best set of ovals that Earth has been able to produce, which were definitely created by wind and water:
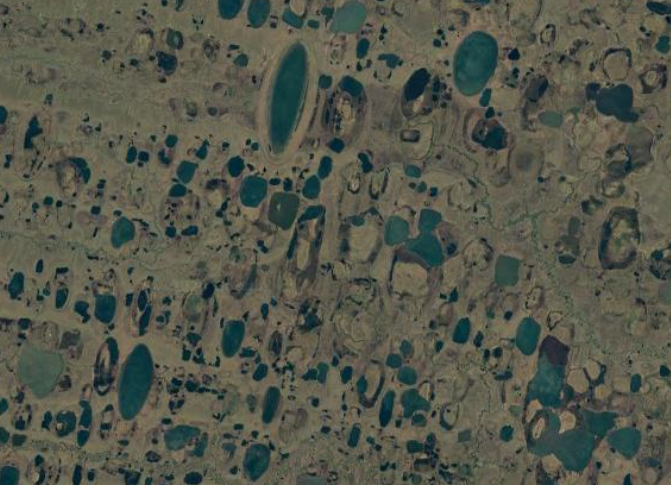
They could be the same thing. But in Siberia there’s a statistical spread, the ellipses don’t have identical eccentricity, and there’s a sense of chaos to the whole arrangement. It doesn’t seem as “mathematically pure” as the Carolina Bays. And they melt together when they touch, they don’t overlap like the Carolina Bays does. Overall, the Carolina Bays are a little suspicious.
So it’s not surprising at all that some people have suggested that the Carolina Bays are actually the effects of an impact that happened at Saginaw Crater. This is consistent with my hypothesis. I took the below image from one of their reports:
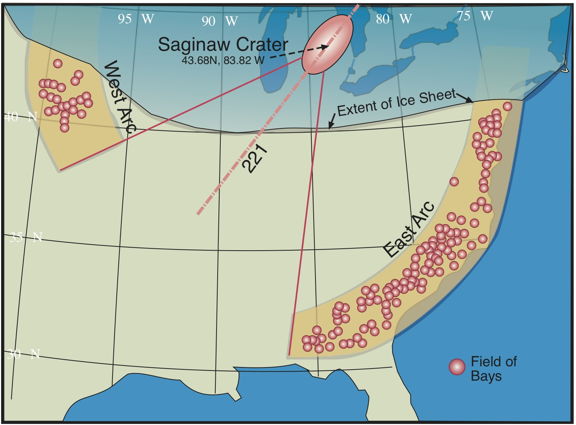
They mean that the Carolina Bays (and Nebraska Bays) were formed, not by cosmic impacts, but by ejected 2nd-hand ice boulders from a cosmic impact. The above image shows the plume of ice boulders that would have been ejected to the sides, if something acted like a giant snow-plow through a glacier. All those rings seem to be where the glacial ice bergs landed, the ones that were plowed out towards the sides! The image is from this website: http://cintos.org/SaginawManifold/Saginaw_Bay/
The problem is that people have investigated the bays tons of times, and have found nothing suspicious. None of the regular impact signs. And while the major axis of the ovals line up with Saginaw Bay, they also point towards the nearest coastline; the direction of the prevailing winds. Which could explain their orientation. And lots of people have done core samples of the stratigraphy of the rims of the bays, and found nothing odd. As far as we can tell, so far, the Carolina bays seem to have been laid down in a steady pace over millennia.
BUT!
Detecting these secondary impacts would be really difficult: Due to the relatively low-speed impact of earth-produced glacier ice into earth-produced sand, there will be very little normal impact evidence. No cosmic materials, and no melted rock. Basically we just throw ice boulders into sand, forming craters, and then we violently shake the sand, causing the craters to shallow out and flatten out. The maximum we should be able to find would be disturbed sand (messed-up stratification) partial and patchy inverted strata in some of the rims, and special warping of the strata underneath the south-eastern elliptical foci. But lots of geologists have looked for such signs and none, to my knowledge, have found such things.
But the bays fit my hypothesis suspiciously well, so if somebody finds evidence for disturbed layers or inverted stratigraphy or warped stratigraphy at the SE foci then please tell me!
Crater formation
Typically, all meteor craters are perfectly circular. That’s because all the energy is dumped into a single point, and the kinetic energy (which is directionless) vastly overtriumphs the momentum (which has direction). So typically it doesn’t matter which way a meteor comes in, it’s gonna leave a circular mark regardless. But if the impactor refuses to deposit all of its energy in a single point, and just continually sheds its energy along a long line, then you’re not going to get a point-like mark; you’re going to get a line-like mark. You’re going to get a trench, not a crater. The trench will slowly widen until the point of maximum energy absorption, and will then narrow off again.
So that’s the most fundamental issue we’re analyzing for each crater: Over how big of an area, volume or distance was the energy dumped? Typically, all impacts happening at oblique angles deposit the energy into a single point, or at least into an area that is so small compared to the energy that it might as well be a point. So typically all impacts produce circular craters, and the main exception are the ones of an extremely shallow angle, or ones that for some other reason shed energy along a line rather than a point.
The impact I propose would have been at an extremely shallow angle (pretty much zero degrees, depending on how you measure it) and therefore it should have a elliptical crater. Which is what we see at Saginaw Crater.
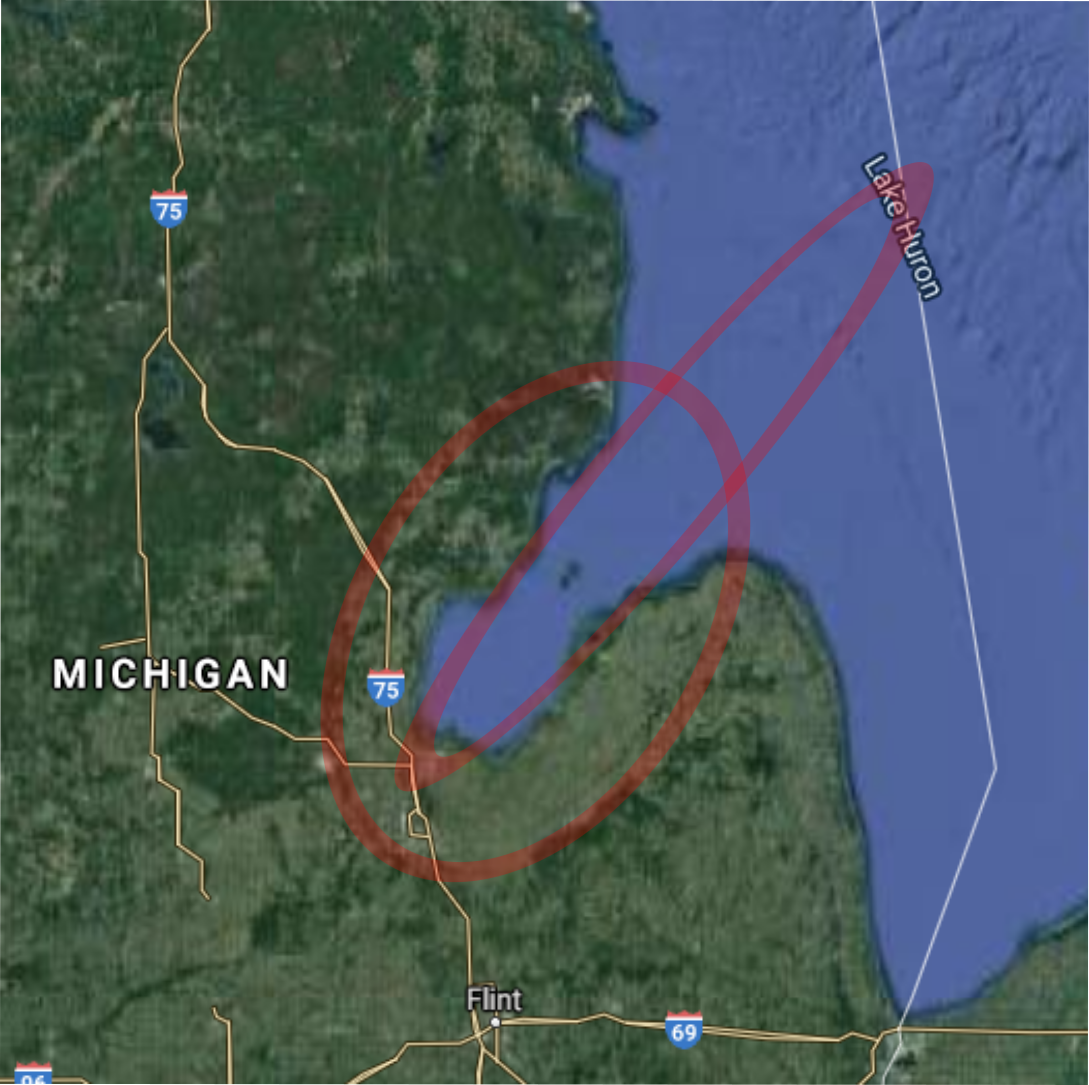
Saginaw Bay (the very elliptical red ring) is 17 km across, and the apparent impact crater (the less elliptical red ring) is 115 km across. Wikipedia has a nice write-up of what crater sizes we can expect from various impact types.
Next steps
I am currently programming a orbital mechanics simulator that shall be able to simulate the solar system backwards in time. I didn’t know how to do that, so I watched a few online university lectures on numeric integrators for gravitational simulation, and implemented the standard approach with a decent integrator and einsteinian physics. And I’ve seeded the simulation with the most accurate input data available (I downloaded huge files from JPL). I intend to use it to backtrace the path of Swift-Tuttle and see if it did indeed pass close to us at that time. I’ve already run the simulator to that time, but I noticed how sensitive it is to minute errors in initial conditions, so I don’t trust it yet. It’s quite tricky to perform a 12 kiloyear simulation accurately. I’ll work for a little longer to improve the accuracy, by various little numerical tricks, and if I end up trusting the results then I’ll post them here.
I also intend to use the simulator to check how the rings would evolve over time, due to the passage of the Moon. I want to measure how long time the rings remain in the required shape before the Moon messes them up too much.
I’d love if somebody who knows impact physics and has access to better tools and data than me could verify the calculations I did. Especially interesting would be if you know how to do a supersonic (Mach 17) air resistance simulation for a loosely-held-together object larger than two kilometers, entering and departing the atmosphere. With and without a solid core.
I’d also love it if somebody who knows climatology would check how much shade would produce the drops in temperature that we’ve measured. That could narrow down the density of the rings. And given that we can narrow down the amount of ice in orbit. And given that, we can narrow down the size of Alpha.
By the way: About rings…
If I have my thoughts in proper order, then rings should be a very common phenomenon. It should happen very often (“often” in geological times speaking) for all planets that have an atmosphere (to slow down a passer-by without destroying it) and at least one large moon (to circularize its orbit afterwards).
Let’s review the planets we got:
- Mercury does not have a moon. So cannot produce rings.
- Venus does not have a moon. So cannot produce rings.
- Earth has both atmosphere and a single very significant moon, so it should be good at producing rings. But it currently has none.
- Mars has almost no atmosphere and insignificant moons, so it should be rather terrible at producing rings.
- Jupiter and Saturn have both very thick atmospheres and plenty of large moons, so both should be excellent at producing rings. I expect that at any point in history at least one of them has rings, and often both at the same time. Currently Saturn has extremely significant rings, while Jupiter has very faint rings.
- Uranus and Neptune have both lots of atmosphere and several moons, so they should be good at producing rings. I expect that at most points in history one of them should have rings. Currently both of them have multiple faint rings.
Therefore I wager that the ring-less-ness of Earth is a very temporary happenstance. I find it exceedingly likely that we will find evidence of more rings at other times in the history of Earth, tilted in different ways, and more or less circularized, compared to the ones I propose here.
That’s all from me. Thank you for reading.